Defining the onset of the Anthropocene
——Twelve sites are considered for defining the Anthropocene geological epoch
Earth’s geological history is divided into chronostratigraphic units that distinguish phases in the planet’s evolution by summarizing complex biotic, geochemical, and climatic changes. Over the past century, many components of the Earth system have changed so much that they no longer occur within the ranges evident during the Holocene—the geological epoch that represents the past ~11,700 years. There are also distinct geological traces that warrant recognition as a new geologic epoch: the Anthropocene. The Anthropocene Working Group (AWG), a task group of the Subcommission on Quaternary Stratigraphy (SQS) of the International Commission on Stratigraphy (ICS), have been working to decide precisely when the Anthropocene began, with a focus around the mid-20th century. The definition will need to identify specific physical properties in sediment layers, or strata, that capture the effects of recent increases in human population; unprecedented industrialization and globalization; and changes imposed on the landscape, climate, and biosphere (1–7).
The definitions of chronostratigraphic units form the basis of the International Chronostratigraphic Chart (ICC) (8) and standardize the geological time scale—e.g., when specific periods, epochs, and ages begin and end and how they can be identified in strata. Protocols established by the ICS to formalize chronostratigraphic units require the definition of a global boundary stratotype section and point (GSSP). This requires the selection of a single reference point for defining the base (the lowermost part) of a chronostratigraphic unit, from which correlation of an isochronous boundary (i.e., one that is the same age everywhere) for the Anthropocene can be achieved globally (9). Given the diversity of geological modification that has occurred in different environments, it is challenging to find a single GSSP that can best represent a global reference for the Anthropocene.
A candidate Anthropocene GSSP site must have at least one primary stratigraphic marker to be used as a reference to correlate the boundary at the selected GSSP site to other locations across the planet (see the figure). These markers include those that indicate a geochemical change—e.g., plutonium isotopes, carbon-14 (14C), and δ15N [based on the ratio of nitrogen-14 (14N)/15N isotopes]—or the appearance of anthropogenic particles—e.g., spheroidal carbonaceous particles (SCPs), which are a type of fly ash only produced by high-temperature combustion of coal or fuel oil (see the photo). Ideally, the site should have precisely datable strata with enough resolution to fix the onset of the Anthropocene to a specific year (10). The strata, mainly extracted as cores at the sites, must represent a complete time record, with no gaps or disturbances in the critical Holocene-Anthropocene boundary interval. A continuous part of the entire core will need to be conserved (for future reference), and the site should preferably be sufficiently accessible that additional cores can be extracted if needed.
Building on many years of previous research (10), 12 candidate sites have been proposed for consideration by the AWG. In addition to the globally important stratigraphic markers (plutonium isotopes, 14C, δ15N, and SCPs), numerous regional markers have been analyzed, including microplastics, heavy metals, and even biological proxies such as the population trends of introduced species (neobiota). AWG voting members are tasked with selecting one candidat e GSSP site.
Two of the candidate sites are in marine sediments—one in the Baltic Sea and one in the coastal Beppu Bay of Japan. For the Anthropocene, suitable marine sediments should exhibit sufficient accumulation rates, be in contact with anoxic bottom waters to limit bioturbation (disturbance by living organisms), and be unaffected by “anthroturbation”—i.e., disturbance by human activities, such as trawler fishing (10). Both the Baltic Sea and Beppu Bay sites contain dark, carbon-rich silts and clays tens of centimeters thick, with the Beppu Bay site also showing seasonal layering. At both sites, these strata lie above the paler, weakly laminated Holocene sediments, and human activity–driven eutrophication (i.e., nutrient enrichment causing phytoplanktonic blooms) is reflected in the prominent textural and compositional changes in the strata along with several other Anthropocene markers, including δ13C (based on the ratio of 13C/12C), δ15N, SCPs, microplastics, and pesticides.
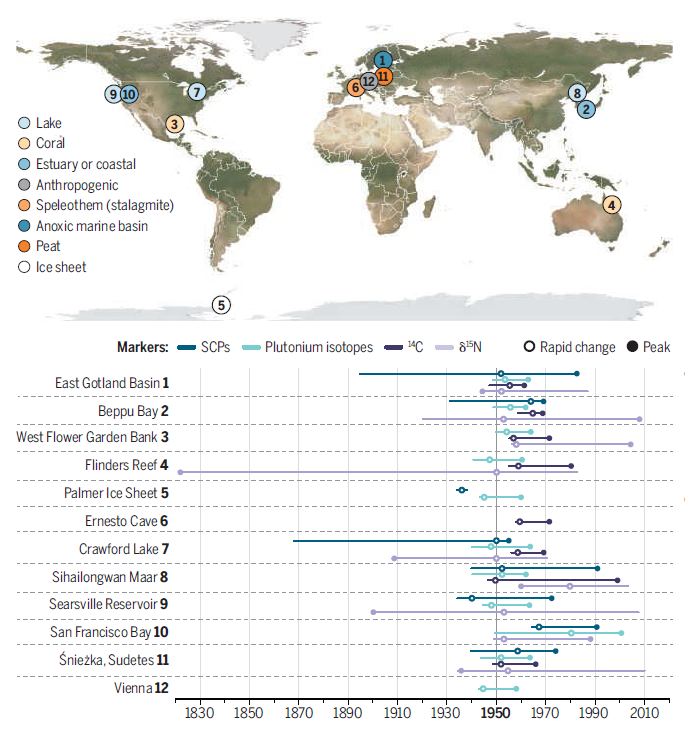
Candidate sites for defining the Anthropocene
Core samples from the 12 candidate sites contain various markers of geochemical change and anthropogenic influence. Globally important stratigraphic markers from each core sample include spheroidal carbonaceous particles (SCPs), plutonium isotopes, carbon-14 (14C), and δ15N [based on the ratio of nitrogen-14 (14N)/15N isotopes]. Lines show the full range of each marker’s presence in the core. The point of rapid change of multiple markers provides chronological correlation between the sites.(GRAPHIC: K. FRANKLIN/SCIENCE)
Two candidate sites are from coral reefs—the West Flower Garden Bank (Gulf of Mexico) and the Flinders Reef (Coral Sea, Australia). Here, the skeletons of long-lived corals preserve visible seasonal growth bands that contain geochemical markers of environmental change, which can be resolved precisely back to the 1750s and 1700s, respectively, allowing for suitable comparison of Anthropocene and late Holocene successions. Both sites show clear effects of increased global fossil fuel combustion driving δ13C to more negative values and proxies indicating warming sea surface temperatures.
Three lake sites have been proposed—Crawford Lake in Canada, which is meromictic (i.e., its water layers do not intermix); Sihailongwan Lake in China, which is a crater lake with a seasonally stratified water column with a lowermost anoxic layer; and Searsville Reservoir in the US, created by a dam built in 1892. The Crawford Lake core indicates ecological effects from indigenous occupation followed by European colonization and shows major ecological changes in its microfossils from the 1950s onward, with carbon and nitrogen cycle perturbations and SCP influx. Sihailongwan Lake is more remote and lacks obvious anthropogenic signals during the Holocene but shows the increased concentrations of soot (particulates formed as a high-temperature condensate), SCPs, heavy metals, and polycyclic aromatic hydrocarbons resulting from fossil fuel burning and industrial emissions. Searsville Reservoir has silts impounded through seasonal storm events with a layer-count chronology tied to local pollution and two earthquake events. At Searsville, SCPs, polychlorinated biphenyls, and pesticides mark the Anthropocene.
The Palmer ice core from the Antarctic Peninsula has also been proposed. Ice cores have previously been selected to define a GSSP for two Holocene subdivisions (11). The length of the core from the Palmer site that corresponds to the Anthropocene is by far the longest among the candidates, at ~32 m. This provides more material to analyze per year, which is important given the low concentrations of some markers in this very remote setting. The core displays seasonally resolvable laminae and records snowfall extremes during the Anthropocene, acceleration of atmospheric methane concentrations, and even small amounts of SCPs.
A core from a raised ombrotrophic peat bog—i.e., one that receives nutrients and water from the atmosphere for growth—near the Sniezka peak in the Sudetes Mountains, Poland, has been proposed. The peat from the site shows an increase of fly ash from thermal power stations, coinciding with the appearance of pollen from introduced plants and perturbations in δ13C and δ15N, respectively, that align with the 1950s.
Three proposals have proved less favorable as the GSSP site: the Ernesto Cave in Italy, the San Francisco Bay estuary, and a section in anthropogenic deposits in central Vienna. The Ernesto Cave site, based on a core of a stalagmite only 4 mm thick that accumulated during the Anthropocene, has 14C and sulfur records that lag behind monitored atmospheric records by 1 to 2 decades as a result of vegetation and soil delaying atmospheric signals reaching the cave. The site was discounted because of this delay. The San Francisco Bay estuary was originally proposed because it has one of the best-resolved historical records of neobiotic species, more than half of which were introduced after 1960. The core records increases in mercury and SCPs in the 1960s and a paleontological succession of five neobiotic species in the 1980s, with their stratigraphic appearances mirroring their known arrival dates. However, because the age of the succession is relatively poorly constrained and the core stopped before reaching the key interval at the base of the Anthropocene, this site was also discounted as a GSSP. Finally, a section of anthropogenic deposits in central Vienna reflects the city’s development and can be dated by artifacts, including debris from World War II. It provides a near-200-year history of pronounced urban changes. However, because of notable gaps during this time interval, the Vienna site is also not suitable as a GSSP.
Together, the 12 sites provide a diverse perspective on the geological reality of the Anthropocene. Although showing regional signals from industrial and agricultural processes with decade-scale variations in timing, global signals provide clear instances of time that can be linked between the sites. Hence, the base of strata marking the beginning of the Anthropocene can be recognized and correlated between all of the sites, whether on land, in ice, or beneath the sea. For instance, one such marker is the acute influx of plutonium isotopes created by a series of aboveground hydrogen bomb test detonations commencing in late 1952 (12). The plutonium provides a sharper global marker than those produced by larger and longer-lasting drivers of Earth system change—e.g., increased burning of fossil fuels and expansion of agriculture—and consequently has been selected by many of the sites as the primary marker.
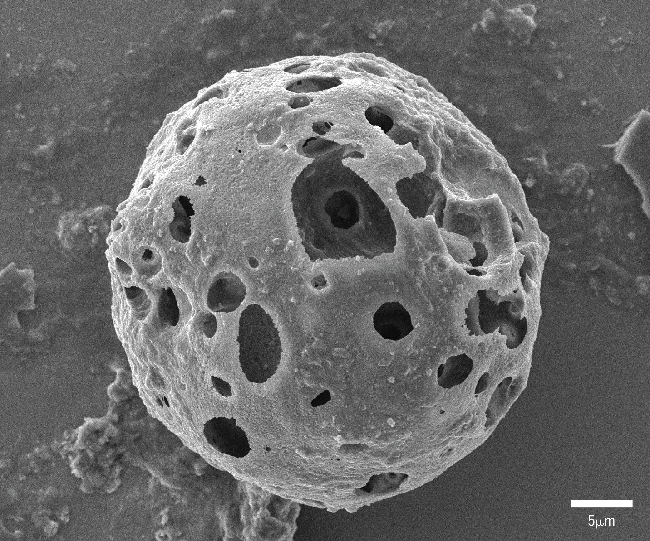
A scanning electron microscopy image shows an ~40-μm-diameter spheroidal carbonaceous particle (SCP) from Crawford Lake, Canada.(PHOTO: SARAH ROBERTS)
The AWG will select their preferred candidate GSSP site based on comparison of the robustness of the core chronology and clarity of physical markers around the onset of the Anthropocene. The chosen site will be used to compose the age name. For example, if the Crawford Lake is chosen, then the current age may be named the Crawfordian Age, which would be the first age of the Anthropocene epoch. The chosen GSSP level at the site will be used to set the precise beginning of the Anthropocene.
The AWG will vote on the site in late 2022, which is only the initial stage in a process. It will be followed by a proposal to be voted on by the SQS, then voted on by the ICS, and finally ratified by the International Union of Geological Sciences (IUGS). Only then will the Anthropocene be formally approved as a component of the ICC. Each of these votes is required to pass by a 60% majority and may fail at any stage, in which case it cannot be subsequently modified for at least 10 years. If approved, the Anthropocene will have a fixed scientific definition with a precise start date that is characterized by a section in the strata with well-defined markers. This will help focus studies on the scale and effect of recent human activity on the planet, contrasting with the relative stability of the preceding Holocene.
(From Science, 17 Nov 2022, Vol 378, Issue 6621, pp. 706-708)
REFERENCES AND NOTES
1. W. Steffen, W. Broadgate, L. Deutsch, O. Gaffney, C. Ludwig, Anthr. Rev. 2, 81 (2015).
2. M. J. Head et al., Episodes 10.18814/epiiugs/2021/021031(2021).
3. C. N. Waters et al., Science 351, eaad2622 (2016).
4. J. Zalasiewicz, C. N. Waters, M. Williams, C. P. Summerhayes, Eds., The Anthropocene as a Geological Time Unit: A Guide to the Scientific Evidence and Current Debate (Cambridge Univ. Press, 2019).
5. J. Syvitski et al., Commun. Earth Environ. 1, 32 (2020).
6. J. Zalasiewicz, C. Waters, M. Williams, in A Geologic Time Scale 2020, F. M. Gradstein, J. G. Ogg, M. D. Schmitz, G. M. Ogg, Eds. (Elsevier, 2020), pp. 1257–1280.
7. M. Williams et al., Palaeontology 65, e12618 (2022).
8. K. M. Cohen, S. C. Finney, P. L. Gibbard, J.-X. Fan, The ICS International Chronostratigraphic Chart (IUGS, 2022); https://stratigraphy.org/ICSchart/ChronostratChart2022-02.pdf.
9. A. Salvador, International Stratigraphic Guide: A Guide to Stratigraphic Classification, Terminology, and Procedure (IUGS and Geological Society of America, 1994).
10. C. N. Waters et al., Earth Sci. Rev. 178, 379 (2018).
11. M. Walker et al., Episodes 41, 213 (2018).
12. C. N. Waters et al., Bull. At. Sci. 71, 46 (2015).